Meteorites are messengers from the depths of time—cast-off fragments of asteroids and comets that formed alongside our sun from raw materials predating our star. But their messages are often muddled by their final encounter with Earth—charred in their fiery plunge through our planet’s atmosphere and contaminated by our world’s ever shifting environment. And unlike a typical piece of lost mail, they don’t come with a return address to reveal their provenance. But what if the scientists wishing to be historians of our solar system’s earliest days could sidestep these problems? Rather than relying solely on the random, scattered chapters of cosmic history meteorites contain, wouldn’t it be better to directly visit space’s most ancient archives—the asteroids and comets—to bring back entire geological books to read?
NASA’s Origins, Spectral Interpretation, Resource Identification, and Security-Regolith Explorer (OSIRIS-REx) spacecraft did just that in 2020, when it dove down to the surface of the near-Earth asteroid Bennu and retrieved some rocks dating back at least 4.5 billion years. Last September it returned to Earth to drop them off. It’s not the first (or second) spacecraft to burgle an asteroid. But it brought back the largest sample to date: a whopping 121.6 grams of pristine material from the solar system’s dawn.
Almost immediately after the sample-return capsule landed on Earth, scientists began their forensic examinations. And earlier this year they presented their first in-depth findings for all the world to see. Their analyses are preliminary, but it seems that Bennu’s original form was shockingly familiar. Billions of years ago Bennu was apparently part of a water-soaked world, one with a beating geological heart and an abundance of prebiotic organic material, now long lost. In many respects, this nameless world could have borne a passing resemblance to the early, lifeless Earth. “Bennu literally carries the building blocks of life within its minerals,” says Louisa Preston, an astrobiologist at University College London.
On supporting science journalism
If you're enjoying this article, consider supporting our award-winning journalism by subscribing. By purchasing a subscription you are helping to ensure the future of impactful stories about the discoveries and ideas shaping our world today.
Billions of years ago Bennu was apparently part of a water-soaked world, one with a beating geological heart and an abundance of prebiotic organic material.
Firmer conclusions are still to come, but already it’s clear that these precious pieces of Bennu harbor immense potential. “What we’re trying to do with these samples is understand how Earth was formed—not just its water, not just its prebiotic compounds, but how Earth itself formed,” says Harold Connolly, a geologist at Rowan University and mission sample scientist for the OSIRIS-REx project.
And it’s not all about our blue-green marble. Some of the sample’s microscopic grains reveal that Bennu’s odyssey began before our sun’s first fires burned, meaning planetary scientists can use it to help answer one of their field’s most monumental queries. “What was that starting mineralogy of the solar system? Where did that dust come from? Did it all come from just one star or multiple generations of stars or different types of stars?” says Ashley King, a meteoriticist at London’s Natural History Museum and an OSIRIS-REx team member. Thanks to the mission’s daring raid on Bennu’s archives, “we’re putting that all together,” Connolly says.
The “Origins” in OSIRIS-REx’s full name refers to the genesis and history of Bennu as a proxy for all other carbon- and water-rich asteroids that have circled the sun these past few billion years. It’s a mammoth undertaking. “We’ve looked at 1 percent of the sample” so far, Connolly says. But this amount is sufficient to begin testing a wish list of hypotheses the team has about Bennu’s life.
A key question: What went into making Bennu’s original (or “parent”) body? Clues reside within its presolar grains, crystals that condensed before the sun existed—“basically, the building blocks of our solar system,” says Pierre Haenecour, a cosmochemist at the University of Arizona and an OSIRIS-REx team member. So far they’ve identified at least two broad categories of presolar grains. Plenty have the chemical signatures of intermediate- to low-mass stars that were in the latter stages of their life; such stars produce potent stellar winds as they age, expelling much of their atmosphere into deep space to create clouds of gas and dust that can be recycled into newborn stars. Other grains hint at a more violent origin. “We do have some presolar grains that appear to have compositions more consistent with what we find in supernovae,” Haenecour says. Altogether these details support the long-standing suspicion that our solar system was seeded and enriched by the explosive deaths of a diverse range of thermonuclear furnaces.
Not long after our sun emerged, worlds began to coalesce around it under gravity’s influence, including Bennu’s unknown parent body. Bennu may exist as a midsize asteroid in a near-Earth orbit today, but the team suspects that eons ago its water-loaded parent first took shape beyond the snow line—a diffuse thermal circumstellar boundary that determines where more volatile substances, including water, can exist as ice around a star.
There is no agreement yet on just how far out Bennu’s protoworld formed. One hypothesis holds that it was not in the asteroid belt between Mars and Jupiter but somewhere farther afield. Key to testing that notion will be the absence or presence of various ices and their residue within the sample. Water ice can exist close to the sun, including within the asteroid belt, whereas frozen carbon monoxide starts to vaporize at a greater distance from it—somewhere in the realm of Neptune.
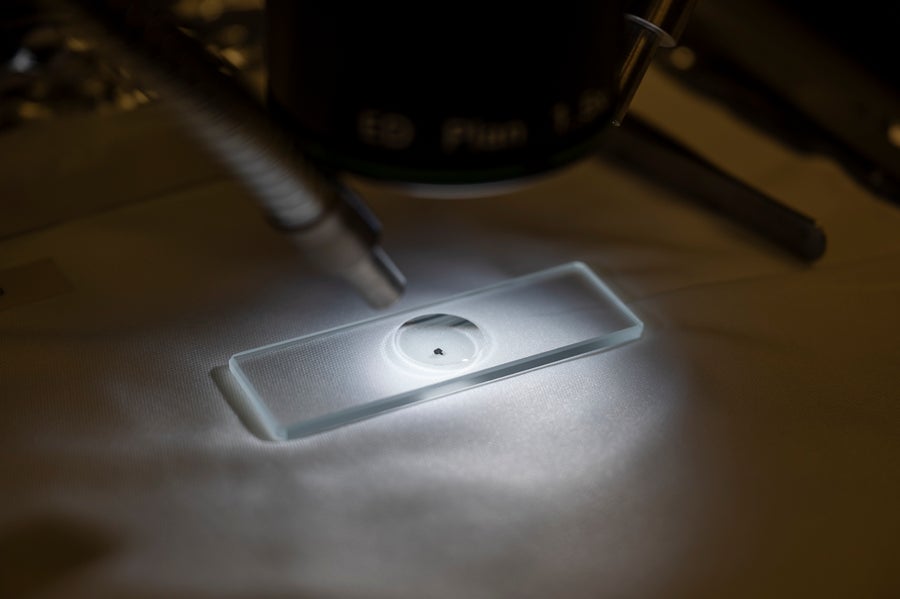
Sourced from a 121.6-gram sample returned to Earth by NASA's OSIRIS-REx spacecraft, a small speck of material from the asteroid Bennu sits on a prepared microscope slide in an exhibition at the Smithsonian's National Museum of Natural History in Washington, D.C.
NASA/Keegan Barber
The array of temperamental chemicals already found in the sample is “consistent with an outer-solar-system origin,” says Kelly Miller, a cosmochemist at the Southwest Research Institute in San Antonio, Tex. Intriguingly, the detection of a soupçon of ammonia, an extremely volatile substance, was also announced at the conference. This could be associated with the asteroid’s organic matter. But if it came from ammonia ice, then “that would push [Bennu’s parent body] out even farther into the outer solar system,” Connolly says—perhaps in or beyond the realm of the ice giant planets Uranus and Neptune.
Wherever Bennu’s parent body formed, it was certainly not in stasis. The sample appears to be packed with clays and other mineral assemblages that are clear signs of dynamic transformations, such as being saturated in liquid water or even having some of that water evaporate to leave behind salts. “Bennu is dominated by materials that are altered by water,” says Sara Russell, a planetary scientist at London’s Natural History Museum and an OSIRIS-REx team member.
Although the water wasn’t scorching hot, it was certainly warm, and its composition might have evolved over time, which suggests that multiple hydrothermal systems were driven by melting ice. That ice melted, at least for a few million years, because the parent body had a toasty geological core heated by the decay of radioactive isotopes. This information indicates that Bennu’s precursor was at least 10 kilometers wide, perhaps larger, Connolly says.
“It’s a beautiful sample,” Russell says. “It’s also not quite like any meteorite in our collection.” For the time being, there appears to be nothing else quite like Bennu, which makes interpreting its mineral makeup a troublesome task—and one aspect of its chemistry in particular has sparked intense debate.
In February the mission team announced the surprising presence of phosphates in the sample. Underneath the icy carapace of Saturn’s moon Enceladus, a geologically tumultuous orb, is a warm liquid-water ocean that contains a range of ingredients essential to life, including phosphorus compounds. After finding phosphates within the Bennu sample, OSIRIS-REx principal investigator Dante Lauretta speculated that the asteroid “may be a fragment of an ancient ocean world.”
“I’m not willing to go there yet, because we haven’t teased out enough of the petrology and petrography to put the story together,” Connolly says. But Bennu is decorated with features that may be linked to surprising geological activity. One of the rock types the spacecraft observed on Bennu looks “cauliflowerlike,” Connolly explains—a smashed-up, squashed-together mess of a sediment-packed rock “that is typically formed in subduction zone areas” akin to those found at Earth’s continental margins and in deep-sea basins. The thought of Bennu’s precursory world having an Earth-like shifting and tumbling of tectonic slabs is tantalizing, to say the least. But these rocks are chaotic and difficult to interpret. “It doesn’t mean that the parent body was tectonically active,” Connolly says.
Currently most scientists are envisioning not so much a geologically hyperactive world as a waterlogged rock with a dynamic youth. “I like to think of it as a big mudball,” King says.
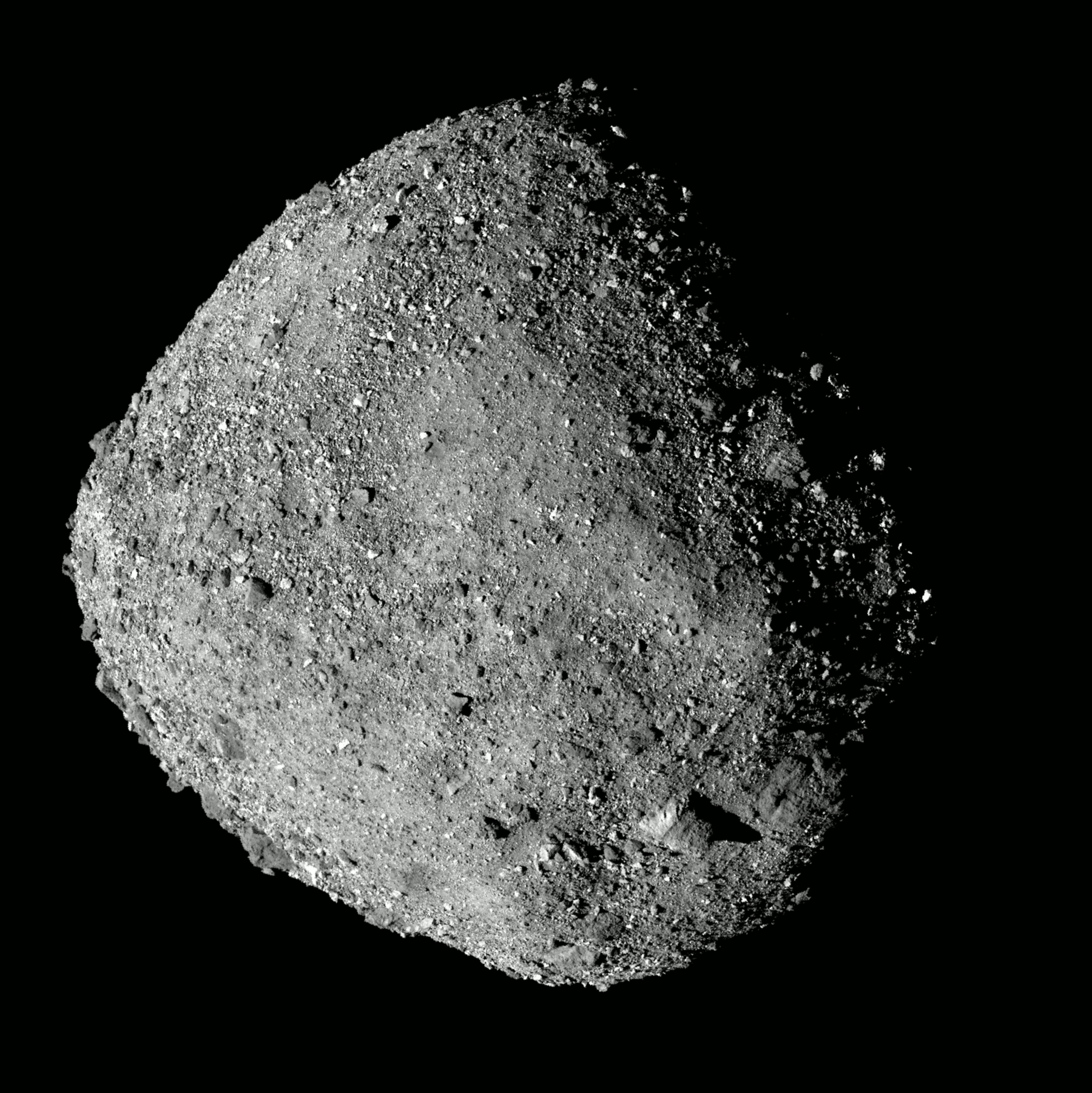
An animation showing the rotation of near-Earth asteroid Bennu, captured over a four-hour period on December 2, 2018 by NASA's OSIRIS-REx spacecraft.
Credit: NASA/Goddard/University of Arizona
That mudball eventually ended up in the asteroid belt, perhaps after being yanked out of a more distant orbit by the gravitational pull of Jupiter. One working hypothesis is that after about three billion years, this parent body was destroyed by a catastrophic collision, liberating the shard we now call Bennu, which then made its way into near-Earth space. That inward migration speaks to a key chapter in the history of the solar system: the delivery of water and prebiotic organic material—carbon-based compounds used by biology—to rocky worlds.
“It’s a long-standing question: Where did Earth’s water come from?” says Richard Binzel, an asteroid expert at the Massachusetts Institute of Technology and an OSIRIS-REx co-investigator. “For a long time we thought it [came from] comets because they’re the most water-rich things we see.” But in recent years analyses of water ice on various comets have revealed its chemical fingerprints to be quite different from those of the water that fills Earth’s oceans.
Conversely, the water found within myriad soggy meteorites is a far closer match to that in our planet’s reservoirs. And what of Bennu? That grand reveal is still some time away, but regardless of whether Bennu has Earth-like water, that perennial query won’t be definitively answered—Earth’s seas and oceans were probably procured from diverse cosmic sources. It’s also possible that their creation wasn’t reliant on asteroids at all; rather Earth’s oceans might have been enclosed within the planet as it formed before escaping to the surface via ancient volcanism.
Then there are the organic compounds. “Biology started off life as chemistry,” Preston says. Even in the exceedingly unlikely event that the OSIRIS-REx sample harbors fossilized alien microorganisms, Bennu won’t provide any concrete answers as to how life got started on Earth. But life couldn’t exist at all without a suite of carbon-bearing compounds such as amino acids. One idea is that these formed in the spaces between the stars before asteroids like Bennu brought these ingredients to our planet.
“We know [that asteroids] can deliver these things to Earth. But the key step is: How did they become life? We need to know that inventory to be able to answer that,” King says. Already the team has identified a long list of organic molecules, including a suite of amino acids, present in the sample. “They even found uracil and thymine—uracil being one of the four nucleotide bases used in RNA and substituted by thymine within DNA,” Preston says. Some of these vital-to-life substances also have primeval inceptions. “Bennu contains organic matter that formed in the interstellar medium,” Ann Nguyen, a planetary scientist at NASA and an OSIRIS-REx co-investigator, said during a conference presentation.
Not all astrobiologists are fixated on amino acids. “I might be a bit of a heretic,” says Cole Mathis, an astrobiologist at Arizona State University, but he isn’t especially interested in abundances of organic matter in Bennu. “It’s not hard to make amino acids,” he says—if you combine nitrogen, carbon and oxygen, “these things are more or less unavoidable.” Asteroids might have delivered them to Earth, but much like the planet’s water, these compounds easily could have formed on Earth without requiring a Bennu-like delivery.
Mathis wants to use Bennu to explore the boundary between chemistry and biology. “There are some molecules that are so complex that only life could have made them,” he says, offering vitamin B12 as an example. He isn’t expecting anyone to find anything like that in the sample. But he wants to find out which molecules can be made by both life and abiotic chemistry and which can be made only by life. “Where should that transition be?” he asks. Bennu, he hopes, will offer hints as to where this boundary lies—because the more baroque an organic compound is, the trickier it is for chemistry alone to make it. Mathis’s query, then, is not about abundance but about chemical convolution: “What’s the most complex individual molecule we can find in these materials?”
Answers to this question and many others are forthcoming. They are hidden within a small pouch of pristine asteroid material awaiting interrogation. Those grains might have cost $1.2 billion to bring to our planet, but they are effectively priceless because they can add context to that famous aphorism: “We are all stardust.” Scientists are now beginning to learn the exact nature, and provenance, of this stardust—the stuff that went into making everything we see, including Earth and ourselves.
Hopes were high when OSIRIS-REx scooped up that sample from Bennu. Already they’ve been surmounted. “The universe was smiling on us,” Connolly says.